Researchers at the Lawrence Livermore National Laboratory (LLNL) have developed techniques that promise to redefine the limits of nanoscale 3D printing. These advances extend the capabilities of two-photon lithography (TPL), a high-resolution additive manufacturing technique capable of producing complex, 3D microstructures, with features two to three orders of magnitude finer than human hair. The techniques also overcome barriers that have limited the functionality of conventional single-photon lithography systems. LLNL’s research aims to uncover design rules for synthesizing photopolymers, with an eye on optimizing TPL for X-ray absorption, which will allow engineers to noninvasively evaluate the physical integrity of deployed 3D printed devices.

Light Curing Systems
To appreciate the significance of these developments, it helps to understand the light-curing process and the factors that determine the resolution of 3D microstructures. Light curing begins with the absorption of light, which initiates chemical reactions that cure the photopolymer. The photopolymer’s solubility determines the curing threshold.For demanding applications, engineers often select a higher curing threshold value to ensure that the strength of the printed structure is sufficiently high. The size of the zone within which the degree of curing exceeds this threshold determines the resolution. When focused light is used for curing — instead of uniform illumination — the system creates a gradient of light intensity. The peak lies at the center of the area, and the intensity progressively decreases as you move away from the center. The photo-initiation process “maps” this light intensity gradient to a gradient of the degree of curing. This gradient ultimately defines the size of the zone in which the curing exceeds the threshold and determines the resolution.
In the case of TPL, the technology involves near-simultaneous absorption of two photons during the curing process, and the amount of absorption scales with the square of light intensity. This means that curing decreases as you move away from the center more drastically for two-photon absorption than for single-photon absorption. As a result TPL delivers finer resolutions.
Tuning for X-Ray Absorption
Although TPL is a robust process when implemented with commercial systems, commercial polymeric photoresists have not proven to be very versatile. For example, the materials commonly used in TPL poorly attenuate X-rays due to the low atomic number of their constituent elements and therefore appear relatively transparent during imaging.This shortcoming poses a problem because as TPL matures, metrology and inspection will play an increasingly important role in the manufacturing process, enabling engineers to ensure that the structure’s geometric form adheres to design specifications. X-ray-based tomography could remedy this by providing a nondestructive inspection technique capable of analyzing stress or defects noninvasively in embedded 3D-printed components or devices.
To address this issue, LLNL’s researchers sought ways to enhance photopolymers’ X-ray absorption while retaining the ability to build structures taller than 200 microns. “Our goal was to deterministically tune the X-ray absorption of the material without losing the ability to print nanoscale features or tall-millimeter scale structures that have sufficient strength,” says Sourabh Saha, one of the team’s lead researchers.
To facilitate the tuning process, the scientists had to discover how to match the “refractive index” of the photoresist material to the immersion oil of the lens so that the laser light could pass through unimpeded. Without “index-matching,” the iterative process of characterizing a newly synthesized photopolymer would be extremely time-consuming because there are simply too many variables to control when tuning material properties. The only way that engineers working with this process could determine if defects were caused by an index mismatch or poor material would be through extensive experimentation.
The researchers were able to tune and increase the amount of X-rays the photopolymer resists could absorb, improving attenuation by more than 10 times over photoresists commonly used for the technique. “We optimized the refractive index (RI) of the photopolymer to achieve the resolution and height metrics and tuned the polymer blend for material strength,” says Saha. “We optimized RI by matching it to that of the immersion medium of the objective. This was performed by mixing the components in such a ratio that their weighted average RI was equal to the target RI. We maximized strength by selecting pre-polymers that have more functional groups per molecule than commonly used pre-polymers.”
It is important to note that index matching also provides the key to printing larger parts, with nanoscale features.
Building Taller Structures
In addition to seeking ways of increasing photopolymers’ X-ray absorption, LLNL’s researchers wanted to be able to print sufficiently strong, tall structures. Previously developed techniques build structures on a glass slide positioned beneath the lens. But because the distance between the lens and the glass slide is limited, only parts 200 microns or less can be printed.
To overcome this limitation, the researchers turned their attention to the dip-in technique. “We opted to use the dip-in mode because it is capable of printing tall millimeter-scale parts,” says Saha. “Tall structures are preferred when post-processing integration into larger structures is desired. Tall structures also simplify material handling and transfer.”
To print tall structures using the dip-in mode, the scientists dipped the lens that focuses the laser’s light into the photopolymer and progressively pulled it away from the printed structure during the layer-by-layer printing process. If index-matched photopolymers are available, dip-in mode is a straightforward technique for printing tall parts with commercially available microscope objective lenses.
Medical Applications
By developing the means to tune photopolymer material’s X-ray absorption, LLNL’s scientists have opened the door for healthcare providers to use X-ray tomography as a diagnostic tool to noninvasively examine the internal structure of 3D-printed objects embedded inside the body, such as biomedical implants, components of drug-delivery systems, joint replacements, and bone scaffolds.The only limiting factor in LLNL’s technology is the time it takes to build the components and devices. The rate of TPL is extremely low today: < 0.1 mm3/hr with submicron features. “We are currently working on scaling-up the process through parallelization to enable the applicability of TPL for 3D printing of consumer-use products,” says Saha.
About the Author
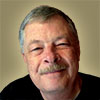
Follow Robotics 24/7 on Linkedin
Article topics
Email Sign Up
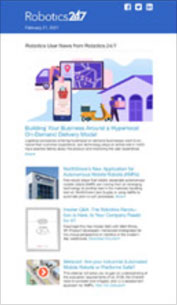
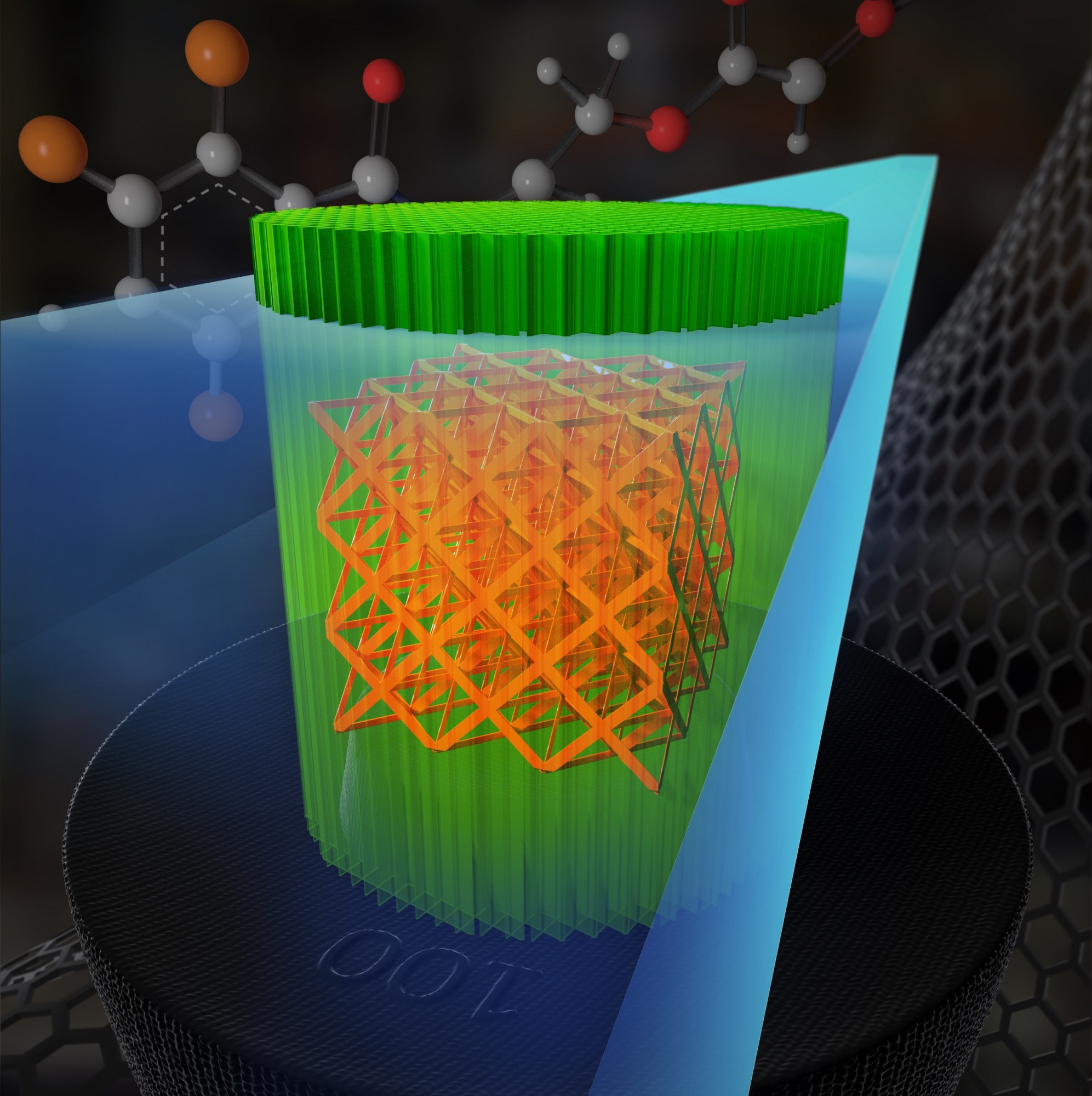